MuBEST lab is currently pursuing the following four research themes:
- Integrative repair of articular cartilage
-
Cell-based tissue engineering (TE) methods represent a promising therapeutic solution for tissue repair. With 3D bioprinting, the next generation TE constructs can be fabricated through precise deposition of biomaterials and cells in 3D space.. The resulting engineered tissues have mechanical properties matching that of the native tissues (Visser et al. 2015). Despite success in fabricating stand-alone engineered tissue constructs, the engineered tissues do not perform well once implanted, with persistent problems associated with integration with host tissues. Currently, research is actively ongoing to improve integrative tissue repair.
One of the most popular testing methods used in the TE community is the so-called push-out test, in which a cylindrical graft is implanted into an annular cartilage host, and the graft is pushed out by an indenter of diameter matching or smaller than the implanted graft (Galarraga et al. 2021, Theodoropoulos et al. 2016). This in vitro graft-host test setup is popular as it mimics the clinical scenario of graft implantation in the joints. However, there is no standardised testing protocol for the push-out test. Considering that articular cartilage, like most soft connective tissues, has time- and rate-dependent material properties, it is not surprising to find a large range of push-out strength (3–221 kPa) in the literature despite otherwise similar experimental conditions. The discrepancies of experimental protocols between different labs also lead to difficulties in meta-analysis of the related studies and therefore limits the progress in integrative tissue repair.
One of the primary research interests of MuBEST lab is to identify the mechanisms governing the biological formation of adhesion between the engineered and the native biological tissues and use the mechanistic models to develop TE methods that can improve graft-host integration. The development of tissue adhesion will be monitored over time through image-based approach driven by multi-photon laser microscopy (Fig. 1). Once developed, the mechanistic models can be used to pinpoint the key regulators of integrative repair, which is of great importance to the tissue engineering and osteoarthritis communities.
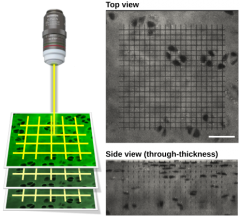
Fig. 1. Three dimensional grid-line imaging approach for intra-tissue strain analysis (Moo et al. 2018)
- Contraction of sarcomeres in a muscle-tendon unit
There are over six hundred skeletal muscles in the human body that govern our daily movement. Skeletal muscles play many roles in our body such as force generation, joint stabilization, body temperature maintenance, nutrient storage, etc. The specific muscle function that MuBEST lab is interested in is the muscle contraction for movement. Muscle force is transferred to joints through another soft connective tissue called tendon. Mechanically speaking, a muscle-tendon unit (MTU) can be considered as a biological motor connected to an elastic spring and a damper through complex serial and parallel arrangements (Roberts & Azizi 2011).
Force is generated in skeletal muscles through sliding interactions between myosin II and actin filaments in a muscle cell. The most basic contractile unit is known as a sarcomere, whose length can be used to infer the force capacity of the muscle. The process of force generation in muscle is governed by both the muscle length and the contraction velocity. In a MTU, the length and velocity at which sarcomeres operate are modulated by the compliance (inverse of stiffness) of the extra-muscular free tendon (Moo et al Plos One 2017). It is known that the mechanical properties of the extra-muscular tendon change with exercise, injury, disease, and aging (Connizzo et al. 2020, Svensson et al. 2016, Freedman et al. 2018). The functional significance of mechanical changes of tendon to muscle contraction is yet to be elucidated.
With the advances in non-linear microscopy, it is now possible to visualise sarcomeres in action within living animals (Moo et al. 2020, Moo et al. Front Physiol 2017). By combining the advanced laser microscopy and mechanical loading system, this group is interested in understanding the changes of sarcomere contraction dynamics as a function of tendon mechanical properties. The findings of this project can enhance our fundamental understanding of force generation and transmission in a MTU, and can have impacts in areas such as treatment and rehabilitative strategies designed for athletes with sports-related injuries as well as function preservation and improvement during aging.
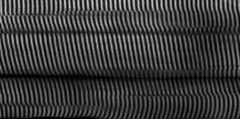
Fig. 2. Sarcomeres visualized under a multi-photon laser microscope. The white bands indicate the anisotropic (A)- band of the muscle where the myosin II proteins are located.
- Relevance of cell shape to tissue integrity and cell viability
Articular cartilage plays an important role in shock absorption and lubrication for pain-free, smooth joint articulation. Cartilage is a resilient biological material with a unique zone-dependent structure and composition. Maintenance of cartilage integrity is done entirely by the cartilage cells, also known as chondrocytes, which takes up < 4% volumetric fraction of the cartilage in human (Hunziker et al. 2002). Maintaining a healthy number of functional chondrocytes is equivalent to having a strong workforce for tissue maintenance and is thought to be essential for healthy cartilage. During early degeneration of cartilage, such as at the onset of osteoarthritis, chondrocytes are observed to change shape, from ellipsoidal to irregular shape with multiple cytoplasmic projections (Karim et al. 2018, Abusara et al. 2021). The origin and the mechanical consequences of the shape change observed in the chondrocytes are not well understood. This group is interested in studying the tissue-cell interaction at the onset of tissue degeneration that eventually leads to the cell shape change. The mechanical impact of the shape change has on mechano-transduction and viability is also of interest. These questions will be answered through a combination of advanced imaging and computation modelling. A mechanistic understanding of cartilage degradation at the cell level can help inform future therapeutic strategies and/or drug development aimed at halting or even reversing the tissue degeneration.

Fig. 3. Cartilage cells have distinct shapes in healthy (LEFT) and mildly degenerated (RIGHT) cartilage. Chondrocytes are in elliptical shape in healthy tissue, but become visibly elongated with cytoplasmic projections in mildly degenerated cartilage.
- Design and development of optico-mechanical systems
Science cannot move forward without reliable measuring tools. In view of the novel scientific questions mentioned above, our group is interested in developing measuring tools to facilitate the quest for scientific answers. For the project related to articular cartilage, this group plans to develop a modular optico-mechanical system that allows for easy assembly-disassembly of the sample chamber to facilitate long-term experimentation. For the skeletal muscle research, an optico-mechanical system that is coupled to an electrical stimulator is desired. This research topic is perfect for students with strong interest in design and hands-on development of measuring system.
We hope you are as excited as us after reading through the overview of our current projects. We welcome any feedback regarding our work. If you would like to join the MuBEST group, please see here. If you would like to collaborate, please contact the PI here.
References
Abusara, Z.; Haider, I.; Moo, E. K.; Miller, S.; Timmermann, S.; Herzog, W. Chondrocyte Morphology as an Indicator of Collagen Network Integrity. Connect Tissue Res 2021, 1–10. https://doi.org/10.1080/03008207.2021.1922398.
Ahsan, T. & Sah, R. L. Biomechanics of integrative cartilage repair. Osteoarthritis Cartilage 7, 29–40 (1999).
Connizzo, B. K.; Piet, J. M.; Shefelbine, S. J.; Grodzinsky, A. J. Age-Associated Changes in the Response of Tendon Explants to Stress Deprivation Is Sex-Dependent. Connective Tissue Research 2020, 61 (1), 48–62. https://doi.org/10.1080/03008207.2019.1648444.
Freedman, B. R.; Rodriguez, A. B.; Hillin, C. D.; Weiss, S. N.; Han, B.; Han, L.; Soslowsky, L. J. Tendon Healing Affects the Multiscale Mechanical, Structural and Compositional Response of Tendon to Quasi-Static Tensile Loading. Journal of The Royal Society Interface 2018, 15 (139), 20170880. https://doi.org/10.1098/rsif.2017.0880.
Galarraga, J. et al. Fabrication of MSC-laden composites of hyaluronic acid hydrogels reinforced with MEW scaffolds for cartilage repair. Biofabrication (2021) doi:10.1088/1758-5090/ac3acb.
Hunziker, E. B.; Quinn, T. M.; Häuselmann, H.-J. Quantitative Structural Organization of Normal Adult Human Articular Cartilage. Osteoarthritis and Cartilage 2002, 10 (7), 564–572. https://doi.org/10.1053/joca.2002.0814.
Karim, A.; Amin, A. K.; Hall, A. C. The Clustering and Morphology of Chondrocytes in Normal and Mildly Degenerate Human Femoral Head Cartilage Studied by Confocal Laser Scanning Microscopy. J. Anat. 2018, 232 (4), 686–698. https://doi.org/10.1111/joa.12768.
Moo, E. K.; Leonard, T. R.; Herzog, W. In Vivo Sarcomere Lengths Become More Non-Uniform upon Activation in Intact Whole Muscle. Front. Physiol. 2017, 8. https://doi.org/10.3389/fphys.2017.01015.
Moo, E. K.; Leonard, T. R.; Herzog, W. The Sarcomere Force-Length Relationship in an Intact Muscle-Tendon Unit. J Exp Biol 2020, 223 (Pt 6), jeb215020. https://doi.org/10.1242/jeb.215020.
Moo, E. K.; Peterson, D. R.; Leonard, T. R.; Kaya, M.; Herzog, W. In Vivo Muscle Force and Muscle Power during Near-Maximal Frog Jumps. PLOS ONE 2017, 12 (3), e0173415. https://doi.org/10.1371/journal.pone.0173415.
Roberts TJ, Azizi E. Flexible mechanisms: the diverse roles of biological springs in vertebrate movement. J Exp Biol 214: 353–361, 2011. doi:10.1242/jeb.038588.
Svensson, R. B.; Heinemeier, K. M.; Couppé, C.; Kjaer, M.; Magnusson, S. P. Effect of Aging and Exercise on the Tendon. Journal of Applied Physiology 2016, 121 (6), 1353–1362. https://doi.org/10.1152/japplphysiol.00328.2016.
Theodoropoulos, J. S., DeCroos, A. J. N., Petrera, M., Park, S. & Kandel, R. A. Mechanical stimulation enhances integration in an in vitro model of cartilage repair. Knee Surg. Sports Traumatol. Arthrosc. 24, 2055–2064 (2016).
Visser, J.; Melchels, F. P. W.; Jeon, J. E.; van Bussel, E. M.; Kimpton, L. S.; Byrne, H. M.; Dhert, W. J. A.; Dalton, P. D.; Hutmacher, D. W.; Malda, J. Reinforcement of Hydrogels Using Three-Dimensionally Printed Microfibres. Nature Communications 2015, 6, 6933. https://doi.org/10.1038/ncomms7933.
Share: Twitter, Facebook
Short URL:
https://carleton.ca/mubest-lab/?p=17