By Tyrone Burke
Photos by Martin Lipman
Making our homes and workplaces more energy efficient is a crucial front in the fight against climate change.
Roughly one-third of the world’s energy is consumed by buildings, and in Canada, where residential and commercial buildings account for more than 20 per cent of greenhouse gas emissions, most of that energy is used for heating. Reducing that demand is one of the cheapest and most effective ways to cut carbon emissions.
But before we optimize a building’s energy systems with renewables such as solar and geothermal power, we need to ensure it has a good building envelope: its roof, sub-floor, windows, exterior doors and walls — basically everything between the climate-controlled indoors and the uncontrolled climate beyond.
“You can have the best energy system,” says Cynthia Cruickshank, a Mechanical and Aerospace Engineering professor at Carleton, “but if you have a bad building envelope, you won’t be able to keep the heat in.”
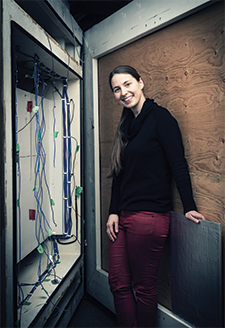
Cynthia Cruickshank
Cruickshank leads the Carleton University Centre for Advanced Building Envelope Research (CU-CABER), a six-year, $5.1-million project, supported by the Natural Resources Canada (NRCan) Energy Innovation Program and the Ontario Research Fund, in partnership with NRCan, Algonquin College, the National Research Council and Alaska’s Cold Climate Housing Research Center.
“Governments around the world are looking for ways to reduce energy use and greenhouse gas emissions from their housing and building stocks,” says Cruickshank. “This problem is most acute in older buildings — the largest segment of the market — which have less insulation and require more heating fuel. Older buildings are also the hardest to renovate.”
To address these challenges, Cruickshank and her team will draw upon advances in super-thin insulation materials, prefabricated construction and panelized retrofits to develop building envelopes that are thinner and cheaper, and new methods for renovating existing buildings with less cost and disruption. Here are some of the tools she is using:
Guarded hot box
“The guarded hot box is essentially a box within a box,” says Cruickshank, cracking open a featureless three-metre cube to reveal an internal chamber that’s wired with sensors, a heater and refrigeration equipment.
One side of the cube is heated, the other cooled. A 1.2-metre by 1.5-metre wall sample is placed between the two. Sensors measure temperature, relative humidity and heat flux — the transfer of energy between the hot and cold sides. Using this data, Cruickshank can calculate a wall’s R value (its ability to resist the transfer of heat).
Cruickshank and her students built the guarded hot box in her lab on campus, but when the new CU-CABER lab opens in 2022 at NRCan’s research complex in Bells Corners, just west of Ottawa, it will have a larger guarded hot box capable of testing three-by-six-metre samples. The new instrument will be able to test walls across a larger temperature range, control the humidity and simulate the use of solar and wind power.
Pressurized spray rack
Beyond the thermal qualities assessed inside the guarded hot box, the air and water tightness of new wall assemblies is of critical importance. To ensure that new building envelope designs and components satisfy air and moisture tightness requirements, and to find out how resilient they are, a pressurized spray rack capable of testing the same sized wall samples will be used.
This piece of equipment will simulate rainfall on building components, and because it will be pressurized beyond standard atmospheric pressure, it will be used to determine whether construction methods and materials can withstand decades of outdoor exposure.
Humidity chambers and guarded hot plate
Building envelopes get wet, and moisture impacts performance. Cruickshank learns how different materials will respond to moisture using humidity chambers and a guarded hot plate.
The glass-doored, two-metre tall humidity chambers have the look of an industrial refrigerator, but instead of cooling air, they regulate its heat and moisture levels. Cruickshank uses them to infuse materials with moisture, then measures the impact this has on performance with a guarded hot plate.
Similar to the guarded hot box, this tabletop machine exposes one side of a material to heat and the other to cold. Sensors measure its thermal resistance, and by comparing the results with dry materials tests, it’s possible to model the impact of wet weather on insulation value.
Temperature baths
The results of Cruickshank’s experiments are used in mathematical modelling. If temperature readings are inaccurate, the results will be too. To ensure that sensors record exact measurements, she uses high-precision temperature baths.
Sensors are calibrated by immersing them in these countertop machines that provide stable temperatures ranging from -35 ̊C to 120 ̊C. Accurate measurements are especially important when modelling a material’s performance over an extended period.
“By exposing the materials to different conditions, we’re essentially accelerating the aging process,” Cruickshank says. “We might be able to simulate five years, and then can curve how the effectiveness of a panel reduces over time.”
Weather station
At Cruickshank’s new lab in Bells Corners, she’ll be able to insert samples directly into its exterior walls. Envelope materials will be exposed to the elements and equipped with sensors that measure their performance.
To understand the exact conditions that materials are exposed to, the lab will have an on-site weather station. This will record precipitation, humidity, temperature and solar radiation, and it will enable precise modelling of weather conditions.
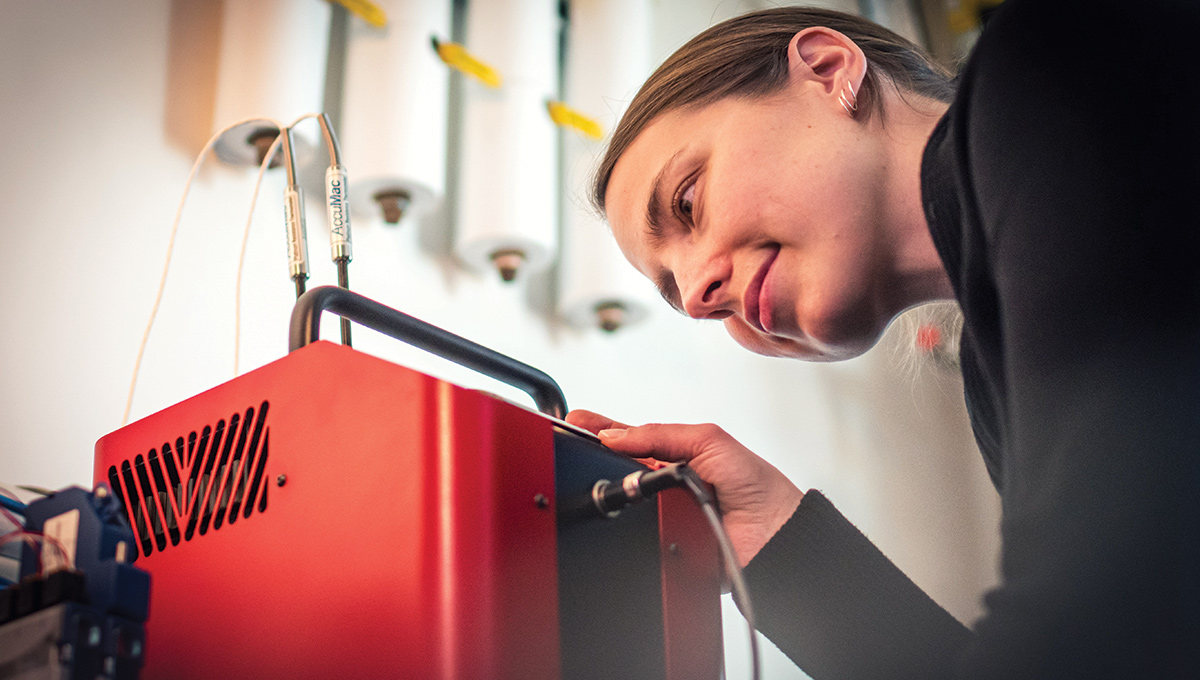
See Her, Be Her
In addition to energy efficiency, Cynthia Cruickshank is passionate about encouraging women to enter science, technology, engineering and math (STEM) fields. “Women can bring a different point of view to a problem,” she says. “It’s about working together and having the strongest team — one that includes both genders.” Here are her suggestions for supporting the next generation of women in STEM:
I Start young
Take girls to STEM-focused activities, such as camps and women-in-STEM events, in their formative years. Head off the discouraging experiences they may have in high school, and show them pathways to STEM careers.
II Raise awareness
Teach girls about women’s achievements in STEM, what they could achieve themselves, and what engineers and other STEM professionals actually do. “Girls should know that engineers solve problems,” says Cruickshank, “and help people.”
III Encourage them
Although many guidance counsellors and parents already promote STEM to girls, it may take more than a couple events throughout the school year to show them that a career in STEM is possible. Three-quarters of students say that their parents have the biggest influence on their career direction.
IV Be a mentor
Female students need support on their journeys toward becoming engineers and support after they’ve joined the workforce. They need champions — people who will help open doors and break down barriers.
V Make women visible
“If I can see her, I can be her,” says Cruickshank, who has a two-year-old daughter. Girls need to have role models and see examples of what they can be in the future. “I would like to live in a world where being a woman in STEM does not make you ambitious or bold — it makes you normal.”
Thursday, January 9, 2020 in Short Reads
Share: Twitter, Facebook