Our research is built on the idea that contaminated habitats are a gold mine for biological diversity.
We are searching for microbes that can be used to recover critical materials and mitigate global pollution.
The biogeochemistry of e-waste (open B.Sc. and Ph.D. positions)
As the demand for consumer electronics has increased in the last decade, so has the environmental footprint associated with their disposal. The global growth of e-waste has created a new and important environment for microbes that can directly interact with toxic and valuable metals that could be recycled. How microbes have adapted to e-waste remains an open question.
We use meta-omics (whole community DNA and proteins) and geochemical analyses to create biogeochemical models for metal cycling in habitats containing e-waste. Our goal is to translate the discovery of novel metal cycling pathways into biotechnology to reclaim metals from e-waste.
We are currently focussed on how microbes affect the fate of valuable metals such as rare earth elements and essential nutrients such as iron. The lab is recruiting B.Sc. and M.Sc. students for research projects starting January 2025. Click here to learn about how you can join the lab!
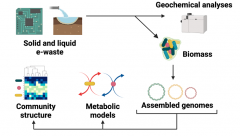
Conceptual diagram of how we characterize microbial communities and metal cycling pathways in e-waste
Microbial corrosion to recover cobalt (open B.Sc. and M.Sc. positions)
Microbial corrosion of iron costs the global economy trillions of dollars by damaging essential infrastructure. Microbes can corrode metals in their elemental state through direct pathways involving redox-cycling proteins and indirect pathways involving redox-active metabolites.
We are interested in leveraging these pathways to recover the critical metal cobalt, which is a major component of battery waste. We combine microbial physiology experiments with analytical chemistry and microscopy to characterize how microbes capable of producing phenazines can solubilize cobalt.
We want to move from mechanistic studies toward validating biological approaches to cobalt recovery in solid waste. The lab is recruiting B.Sc. and M.Sc. students for this research project starting September 2025. Click here to learn more about the positions available!
The impacts of contaminant mixtures on microbial communities
This research examines how microbial consortia interact with contaminant mixtures in landfills. Landfills contain diverse communities of microbes that have adapted to live alongside hazardous chemicals. How the toxicity of these mixtures affects microbial community composition and selection for pathways that could be used for environmental remediation is poorly understood.
This project is in collaboration with Dr. Jordyn Broadbent and Dr. Derek Smith from Environment and Climate Change Canada. We combine metagenomic sequencing (whole-community DNA) with analytical chemistry and bioinformatics tools to connect environmental toxicology and microbiology data as part of this work.
The outcomes of this project will directly inform risk assessment and management of landfill leachate in Canada. We are recruiting an M.Sc. student to lead this project starting September 2024. Click here to learn more!
The biochemistry of plastic cycling in solid waste
Identifying different plastics in solid waste remains a major challenge for recycling companies because of the different plastics used in consumer goods. Many plastics contain toxic additives such as flame retardants that negatively impact human health when released into the environment. Being able to characterize plastics and plastic additives rapidly is critical to sustainable plastic reuse.
This collaboration is with Dr. Yaxi Hu’s FACT lab in the Department of Chemistry at Carleton. We focus on developing new analytical chemistry methods using Raman spectroscopy and machine learning algorithms to identify plastics and plastic additives in plastic waste. We are exploring how these tools can be used to monitor plastic biodegradation in electronic waste.
Microbial solutions to “forever chemicals”
Per- and polyfluoroalkyl substances (PFAS) are anthropogenic compounds used in numerous industrial processes and consumer products. Thousands of PFAS are eventually released into the environment where they can persistent, bioaccumulate, and exert toxicity. There is a pressing need to understand what biochemical processes control the fate of these compounds in the environment and our bodies.
This collaboration is led by Dr. Amy Rand from the Department of Chemistry at Carleton. We are coupling genetic sequencing approaches and mass spectrometry tools to characterize novel mechanisms for PFAS cycling in the environment and human gut. We aim to use these findings to develop bioremediation strategies for these “forever chemicals” and inform risk management strategies to mitigate PFAS toxicity.
Arsenic bioremediation in groundwater
Understanding the biogeochemical processes that control arsenic’s fate in the environment is critical to mitigating potential health issues linked to arsenic exposure. Microbes can control arsenic’s mobility and toxicity through dedicated detoxification strategies and metabolic pathways. These adaptations can be used in bioremediation strategies to limit arsenic’s mobility in groundwater systems.
This research is an ongoing collaboration with Imperial Oil Resources Ltd. and Dr. Laura Hug at the University of Waterloo leveraging microbial adaptations to arsenic to support bitumen extraction. This research combines whole-community DNA approaches, geochemical analyses, and microbial cultivation experiments to explore biological approaches for immobilizing arsenic in aquifers using microbes that naturally occur in these habitats.
Signatures of early life on Earth using tungsten
When life originated on Earth remains one of the most fascinating questions of our time. Biological pathways that control elemental cycling via enzymatic activity offer a way to search for preserved biosignatures that give us clues to help answer this question. These same approaches can also be used to search for life on other planets that harbour the same elements.
This research focuses on developing multidisciplinary approaches to determine if tungsten isotope fractionation can be used as a biosignature in habitats representative of early Earth. We aim to measure these biosignatures in Earth’s oldest rocks and better understand how signatures identified in model microbes can be used in the search for life beyond our planet. This collaborative project is funded by the New Frontiers in Research – Exploration fund, led by Dr. Hanika Rizo, and spans the Biology, Chemistry, Earth Sciences, and Engineering departments at Carleton.