Novel Plasmonic Nanomaterials: Design, Properties, and Application
In this research project we:
- synthesize metal nanocrystals of various nature.
- assemble them in two and three dimensional plasmonic structures.
- fine tune their optical properties (refractive index sensitivity, Raman and fluorescence signal enhancement).
- apply in fiber based sensor devices, solar cells, and for imaging.
When nanoparticles of coinage metals are illuminated with light collective oscillations of conducting electrons in such nanoparticles can be excited, called localized surface plasmon resonances. These plasmon resonances are responsible for bright colors of colloidal solutions of nanoparticles, traditionally used in glass staining and art. Plasmons enhance electric field near the surface of such nanoparticles more than million times, are very useful for ultrasensitive molecular detection, and can also help guide and manipulate light at the nanoscale, needed for next generation opto-electronic devices and solar cells. Besides, over the past 30 years gold and silver nanoparticles have been extensively used for biomolecular imaging, labelling, and sensing. Traditionally, however, only spherical nanoparticles of gold or silver have been utilised. Although such nanoparticles are easy to fabricate and manipulate, their plasmonic properties are rather generic due to isotropic shape, which limits control over plasmon resonances.
We have recently discovered that plasmonic properties of cubic nanoparticles are much more attractive and useful in the above mentioned applications. For such nanocubes the number of plasmon resonance modes increases, the degree of electric field enhancement increases, and shape anisotropy enables effective assembly of such nanocubes in two and three dimensional structures. Development of novel plasmonic nanomaterials based on self-assembly of metal nanocrystals constitutes the long term objective of our research program.
Antimicrobial Peptides: Molecular Mechanisms of Action
In this project we investigate new potent antimicrobial peptides in order to determine the effect of small modifications in the primary structure on their physicochemical properties and antimicrobial activity with the objective of creating safer and more efficient antibacterial agents.
The continuous use of antibiotics has resulted in multi-resistant bacterial strains. Finding alternatives to synthetic antibiotics is therefore of great importance at the moment. Antimicrobial peptides (AMPs) are components of the innate defence system of many organisms and display potent antimicrobial activity against a variety of microorganisms by direct action (disrupting bacterial cell membrane) or by modulating immune system response. AMPs are relatively short, usually highly amphipathic, cationic peptides. Although some AMPs have been found to interact with intracellular targets, it is believed that interactions between AMP and the cell membrane play an important role in the activity of the peptides. Despite the fact that natural AMPs have been studied for a considerable period of time, only several peptides have advanced into phase 3 clinical trials, but none have been approved for medical use. This is mainly due to the relatively high rapid in vivo degradation and high costs of production. Thus a number of synthetic AMPs have been derived and studied to circumvent these problems.
We use a combination of biophysical and biochemical techniques such as scanning probe microscopy (SPM), UV resonance Raman (UVRR) spectroscopy, Circular dichroism (CD), Langmuir-Blodgett (LB) monolayer technique, calcein leakage assay and minimum inhibitory concentration (MIC) assay. This approach will provide new and unique experimental data that will reveal more details on the molecular mechanisms of AMP action in general, and will help us develop safer and more efficient medications.
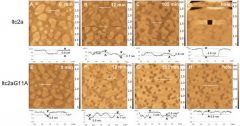
AFM imaging the kinetics of lipid bilayer disruption by latarcin 2a (ltc2a) and derivative latarcin 2aG11A.
High Resolution Imaging of Biological Samples
Basic Scanning Probe Microscopic (SPM) techniques are classified by the type of probe used: scanning tunneling microscopy (STM), atomic force microscopy (AFM), near field scanning optical microscopy (NSOM). NSOM enables optical measurements with nanoscale spatial resolution. It combines the resolution of AFM with the sensitivity, specificity and flexibility afforded by optical techniques. A sub-wavelength-sized light source illuminates a sample in the near-field (at a distance much smaller than the wavelength of light) and induces an individual optical response that can be collected in the far field. A variety of steady-state and time-resolved spectroscopic techniques can be used to analyze the sample. In our group we focus on combining NSOM with Raman and surface-enhanced Raman scattering (SERS) spectroscopy for chemically specific imaging of membrane- bound small drugs and peptides with nanometer-scale spatial resolution.
For more details of the research, see our recent publications.